-
IN QUEST OF INFINITY – 23
By Prof. G. Venkataraman
Loving Sai Ram and greetings from Prashanti Nilayam. We have reached an important milestone in our wanderings that have stretched almost two years now! Amazing is it not? Perhaps you are wondering how much longer it would be; my guess is that may be it might be a few more months before we get a distant glimpse of INFINITY, and after that, you would have to explore on your own; that is because I would be leaving you all alone, having done my job as a guide!
OK, where do we stand now? To refresh your memory, I have given you a broad-brush introduction to Quantum Mechanics [QM], and also to Born’s probabilistic interpretation of the wave function – this I did in the last couple of issues. I am sure when Born first gave his interpretation, few understood what on earth he was talking about.
That of course did not prevent many from commenting extensively on Born and his ideas – you know, it is always easy to talk nonsense about everything on earth, pretending to be very knowledgeable about it all. People do it all the time about everything, from politics and economics to science and spirituality [I hope you do not have that impression about this series!]. Anyway, the point I want to make is that a few people did reflect deeply about the implications of Born’s interpretations and spent a lot of time worrying about it. In this issue, I wish to tell you a bit of that story.
Theories Reflecting Reality
Before I do that, some preliminaries are necessary. Firstly, let us ask what exactly a theory in Physics is supposed to do. I mean I talked a lot about Classical Mechanics that got a kick-start from Galileo and Newton and all that, and was subsequently honed to a fine art by many, including giants like Lagrange in France and Hamilton in England. What was the big deal about all that they did? Sure they gave a lot of formulae and methods to do complicated calculations that help us even today, especially in engineering. But that apart, was there anything basic about what they did?
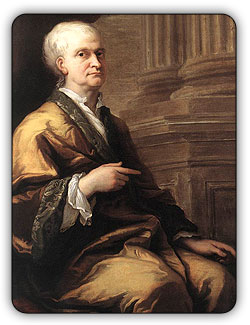 |
 |
|
Sir Isaac Newton |
|
Galileo |
Yes there was, and that is what I wish to discuss in this issue. Let me start with what a theory in Physics is supposed to do. Basically, it is supposed to give some kind of a picture of “physical reality”. OK, but what is this “physical reality” one is talking about? It turns out that the answer to this question is not so simple. For centuries, physicists believed that physical reality underlying their complicated equations, like the rainbow, the Sun, the Moon, etc., related to how we perceive them.
That seemed OK, and why not? But if you think about it for a moment, you would realise that entities like the rainbow, the cloud, snake, frog etc., are entities that the senses perceive, after which a certain impression related to these entities is created in the human brain. Thereafter, every time we think of say, a stone, some kind of an image pops up before our mental screen and we feel perfectly fine.
Let us say that a teacher says to his students, “Boys, today I am going to teach you how to calculate the trajectory of a stone that is thrown in a particular direction and with a particular velocity.” When the teacher says that, all the students immediately form some kind of a picture in their minds, and thereafter, when the teacher writes equations on the board, and draws sketches of the trajectory, it all seems to fit and hang together. At the end of the class, the students walk away feeling they have understood it all, that is if they were not sleeping! This is the way, a contact was made between what we all believed was physical reality and the equations discovered by the giants of Classical Physics.
I should mention here that Classical Physics dealt with matter on a macro scale and that too when it was not moving very fast, meaning that the speeds involved were a tiny fraction of the velocity of light. As you might recall from some of the earlier issues in this series, the extension to the case when the speed approached that of light was made in 1905 by Einstein, via his famous Special Theory of Relativity.
So what was the reality that stood revealed thanks to all this work up to say the beginning of the 20th century? As far as the founders of Classical Mechanics was concerned, starting from Newton all the way to the great masters like Hamilton in the 19th century, matter was a continuum that could be compressed as well as stretched. When heated, solid matter could melt and become a liquid.
If heated still further, it could become a gas; in all these three states, matter was held to be a continuum. However, when issues related to heat were considered, it seemed that the continuum hypothesis had to be given up and matter treated as if it was made up of atoms.
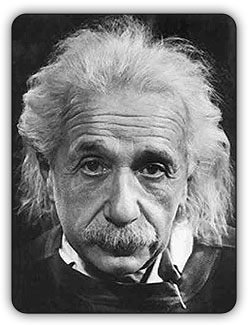 |
 |
|
Albert Einstein |
|
James Clerk Maxwell |
Atomic Discovery
Maxwell did this in order to develop his kinetic theory of heat. He did not know what atoms were like but that did not stop him. For him, atoms were like tiny billiard balls that moved fast, that could collide and get scattered, etc. The collision could be treated very much like billiard ball collisions via Newton’s equations, and this was how Maxwell linked his work on heat to mechanics. Maxwell still retained contact with Classical Physics, except that he abandoned the continuum picture of matter.
Maxwell’s work was extended and given a much firmer footing by Boltzmann of Austria. So complete was his theory that it is used even to this day in certain situations. For Boltzmann, matter HAD to be made up of atoms, even though he did not know what atoms were made up of. Strangely, even though it was as late as the turn of the 19th century, many physicists of those days objected to atoms, although chemists were not only quite comfortable with the idea of atoms but had done a tremendous job of classifying them and organising their family tree in terms of the famous Periodic Table.
At a scientific gathering where Boltzmann presented his findings, he was ridiculed by some, including a prominent man of those times named Ostwald. Boltzmann felt so humiliated that he committed suicide, a great tragedy because even as he gave up his life, the concept of atoms was not only getting firmly established in Physics but was in fact opening the door to a whole new universe one might say – but then, that is life!
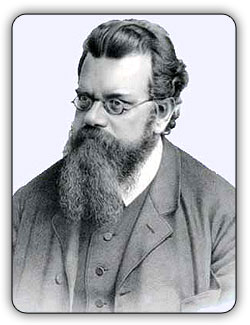 |
 |
|
Ludwig Boltzmann |
|
Wilhelm Ostwald |
I have no doubt gone over all this before but there is a reason why I am repeating some of it now. You see, starting from around 1900 or so, scientists were not only beginning to learn more about the atom but also its internal constitution, that is to say what it was made up of. They learnt that the atom had a tiny nucleus where all the positive charge was concentrated, and that around this nucleus were distributed in some manner the electrons, also very tiny.
Bohr then came along and gave a picture of the atom and also a mathematical model for describing the atom and some of its properties. The maths was quite elementary but the concepts Bohr invoked were far reaching. Thanks to him, physicists realised that the atom could NOT be described using the equations of Classical Physics, that is to say the equations of Newton etc., that had till then ruled so powerfully for centuries in fact. As Americans would say, the atomic world seemed to be a whole new ball game.
|
 |
Bohr's atomic model |
|
Bohr understood it was indeed a new game, but did not uncover the rules of that game; he merely discovered the key to the lock. As I described earlier, the lock was opened by the trio Schroedinger, Heisenberg and Dirac, after de Broglie had pointed out where the lock was! All that might be a rather colloquial way of stating crucial historical facts, but the fact is that it was de Broglie who first suggested that like light, matter too might have a dual aspect.
Then came the trio I mentioned, and at the end of it all, say by 1927 or so, one had all the equations for dealing with matter on a microscopic scale, provided the velocity was small compared to that of light [technically, this is called the non-relativistic limit]. The extension to the relativistic domain came soon after [by the way that extension was due to Dirac], but that detail is not important for us presently.
What did the Discoveries Point To?
OK, one had equations using which one could now make good and reliable calculations but what did they mean? The answer to that was far from clear. By the way, during those early days, many important discoveries were made simply working these new equations; as someone said, those were days when second-rate physicists could do first-rate work! But what was the nature of the “physical reality” hiding behind these equations? That was the question bugging many top minds.
To give you a flavour of the concerns people had in those days, let me mention a few points. Consider for a moment, the duality of light and matter. Experiments seemed to show that light seemed to behave like waves in certain experiments while in other experiments it manifested as a particle. Matter too showed the same kind of duality.
Naturally, people were puzzled. In the case of light, for example, they asked: “Is light a wave or a particle? Or is it both? Or is it one thing at one time and another thing at a different time?” No one knew. So frustrating it was that it used to be said that light behaved like a wave on Monday, Wednesday and Friday, as a particle on Tuesday, Thursday and Saturday, and observed the Sabbath on Sunday!
 |
|
|
David Bohm |
Then came the wave function Ψ of Schroedinger, a complex quantity with an imaginary part, adding to the confusion. As I described in the last issue, Max Born no doubt clarified matters to some extent with his probabilistic interpretation, but by and large, even though most people could work the equations and get results that could be compared with various experiments, where understanding “physical reality” was concerned, they were no wiser than school kids!
The Mysteries of Quantum Life
It was really a very strange situation that very much bothered the masters. The mood is fully reflected in the following quote from David Bohm, who came later and devoted a lot of his time to understanding the philosophical implications of quantum mechanics. He says:
Quantum mechanics says that Nature is unintelligible except as a calculus, that all you can do is to compute with the equations and operate your apparatus and compare the results.
I am sure all this might leave you a bit bewildered, but that is to be expected. After all, even the pundits were foxed when they first had to deal with QM (quantum mechanics), and the funny thing is that even today, not many really understand what exactly are the philosophical implications underlying QM. This being the case, I obviously cannot quite enlighten you. However, there are a few things I could do and they are the following:
- I shall give you a glimpse of debate that raged during the early days, focussing in particular on the arguments that raged between Einstein and Bohr. It was almost like a gun fight in a Western movie! But no violence; only vehemence in the arguments!!
- I shall then fast-forward and tell you about a terrific surprise that emerged in the seventies.
- And wrap it all up with some words where does all this leave us.
[I might not be able to do all that in this issue, but at any rate, that would form the theme of the next couple of issues.]
All the above might leave you a bit confused – but you should not worry about that. What would happen at the end of it all is that we would slowly be able to step out of the physical world [that seems so real to us!] and enter the world of meta-Physics, from where one could glide to the realm of Vedanta without even being aware of it! In short, the journey ahead is exciting and heady, even if we pass through territory we cannot quite comprehend. With that preamble, let me move on.
|
 |
The wave function or 'eigenstates' for the hydrogen atom |
|
I shall start with some remarks by Max Born, who, if you remember, actually started all this business of probabilistic interpretation of QM. Soon after he gave his view, Born said that while Schroedinger gave a method of calculating the stationary states of a quantum system, he did not say in which state the system would be found if an observation is made. The wave function associated with the stationary states could be computed using Schroedinger’s method, and let us designate them say as, Ψ1, Ψ2, Ψ3, Ψ4, Ψ5, Ψ6, etc. In German terminology, these are also called eigenstates.
OK, let us say there is a hydrogen atom. If one solves the Schroedinger equation for the hydrogen atom [I said something about this type of exercise in an earlier issue], then one would be able to obtain the eigenstates of the hydrogen atom. These were earlier called by Bohr as allowed states. Suppose there is a hydrogen atom in the lab.
The question could be asked: “In which of the allowed states is this particular atom? Can QM give an answer to that?” The short answer to that question would be: “You cannot give any answer to that question, except to say that in general, the atom would be in a state Ψ say, which is some kind of a mixture of all the allowed states.” This can be formally expressed by writing:
Ψ = aΨ1 + bΨ2 + cΨ3 + dΨ4 + eΨ5, ……..
where a, b, c, d, e, …. etc., are numerical coefficients. As you can see, the state Ψ is a mixed state, that is, it is some specific mixture of the eigenstates of the hydrogen atom. That is all that can be said, nothing more nothing less.
Suppose one now says: “OK, let me find out what exactly this Ψ is. How do I do it? By making an observation of course.” So you make an observation. You know what you would find? Something you would not expect at all. You would find that the atom is not in the state Ψ, but in some other state, which could well be one of the eigenstates mentioned earlier. That is, the process of making the observation disturbs the atom, causing it to jump from the state Ψ to may be Ψ1, Ψ2, Ψ3, or something like that, or may be even some other mixture – who knows!
This was a totally new experience in the world of Physics. In Classical Physics, when a system made a transition from one state to another, one could observe what state the system was in to start with, and, based on the equations of Classical Physics, predict precisely to what state it would go. In QM it was totally different. One could a priori not say what [quantum] state the system is in to start with. If we try to determine that state via an observation, the system fools us by jumping to some other state! It seems as if quantum states do not want to be observed!
 |
|
|
Max Born |
Suppose we insist on making an observation; the system jumps to some state; which one? We cannot say for sure; all we can do is to make some kind of a probabilistic forecast. That was the discovery Born made.
By way of stressing all this, Born once commented on what would happen if two particles collided, like say two [quantum] billiard balls. Prior to the collision, the two-particle system would be in a particular state, and after the collision it would go to another state; which one? Responding to that question, Born says:
One obtains the answer to the question, not “what state after the collision” but “how probable is a given effect after the collision” …I myself tend to give up determinism in the atomic world.
The exit of determinism - that is the crucial point about QM. You see, in Classical Physics, there was determinism. If you applied a force on a system that is well defined etc., then you could predict precisely what would happen as a result of the application of the force. Let me put it this way.
Suppose one were to fire a classical cannon ball, in a particular direction and with a specific velocity, one could then, using the equations of Classical Physics, calculate precisely where the cannon ball would hit the ground.
There was only one unique answer, because the entire process was governed by laws whose underlying principle was determinism. If there was a cause, then the nature of the effect produced was precisely predictable [assuming one has complete information about all the relevant parameters].
Einstein Rejects QM: ‘God does not play dice’
In QM, it seemed that was not the case. When a quantum cannon ball is fired by say a quantum gun into a world ruled by QM, then one cannot say for sure where the ball would hit the ground. One could only talk of probabilities, and this probabilistic nature is built into the very fabric of QM. It was, as Einstein put it, that God was playing dice; and that was a notion he intensely disliked. In short, it all started with Einstein saying that he did not trust QM because there was something about it that was intensely distasteful to him.
|
 |
YIKES! QM left a bad taste in Einstein's mouth |
|
His attitude was:
“How can Nature be non-deterministic? That is inconceivable and I totally reject that idea. I don’t care what you fellows say but to me, God does not play dice! I don’t know about you but I am very clear about it!”
That is not an exact quote but basically that summarises Einstein’s reaction to QM, at least in the early stages.
However, it did not end there, with Einstein simply rejecting the notion of QM and others murmuring, “The man has lost his touch, you know!” Einstein was too important a figure and one could not have him objecting to the new and revolutionary physics that was strongly emerging. Such a deep division on so crucial a matter would be tremendously damaging to the progress of Physics – so thought many, and they began to think of ways of convincing Einstein that QM was not a lot of hocus-pocus but a mechanics that did deliver the goods.
If it did that, clearly Nature was sending a message; it was the duty of all Physicists to try and decode that message. Taking that view, Bohr decided to engage in a public debate with Einstein. That story forms a fascinating and an important chapter in the history of QM, and that is what we shall turn to next.
Epistemology and Ontology and the Throw of a Coin
Before I get to the folklore part of the historical Bohr-Einstein debate, there are a few technical points I should maybe get across. To start with, there are two words I should introduce you to. They occur frequently in discussions on philosophy, and they are: Epistemology and Ontology. The former word means the theory of knowledge; in our context, it simply means OUR knowledge or conception about a physical system. Notice the stress on the word “OUR”. The emphasis is laid because our knowledge about the system might in fact be quite different from what the system actually is – but that is an altogether different matter.
Turning to the other word, the dictionary meaning of ontology is: the branch of philosophy that deals with the nature of being. In our context, it refers to the nature of the system, as it actually exists. Roughly speaking, epistemology deals with knowledge built up from observations, whereas ontology refers to the attributes the system has, independent of whether one observes it or not.
Perhaps a simple example would illustrate the difference. Suppose you toss a coin. Everyone would say that whether the outcome is heads or tails is a matter of probability. From a practical point that is indeed the case. However, the question can be asked: “Is it not possible to calculate, using the full machinery of classical mechanics, what the outcome would be?”
Yes one can do that, but for making such a deterministic calculation, one must have all kinds of details about the coin, including its weight, shape, distortions to the shape due to handling, dust accumulated on it, full details about the impact made by every molecule of air colliding with the coin as it is tossed up and falls down, the breeze blowing and so on. Obviously there is just too much detail; and even if we know them all, the calculations would be horrendous; simply not worth wasting time on doing it.
So, one prefers to remain ignorant about the details, and when we lack full knowledge of the circumstances and the parameters involved, we can only make a guess about the outcome; and that is how we end up saying there is a 50-50 chance it would be heads and things like that.
To put it in nutshell, the system is intrinsically deterministic, but thanks to our ignorance, OUR knowledge of the system ends up being probabilistic. We thus have here a case of ontic determinism but epistemic indeterminism! I hope you are able to follow that. Barring such cases, Classical Physics is all about determinism, where outcomes can be calculated in a deterministic rather than probabilistic manner. Got that?
Following the Rules of Classical Physics
A few more moments on certain aspects of Classical Physics, in order to appreciate how Quantum Physics differs drastically. The first thing we note is that determinism is an important feature of Classical Physics. Thus it is that we have what is called Causality – there is a cause, which produces an effect. In other words, determinism and causality are two sides of the same coin. Having grown up with Classical Physics, Einstein was a strong believer in determinism and causality; QM rocked that boat, and he naturally became very disturbed – that is the story we now are preparing for.
To continue, measurement forms an important aspect of QM. It is useful therefore to understand first what exactly making a measurement means in Classical Physics; that would help us to appreciate better how different things become, once we enter the quantum world. Two important assumptions made in Classical Physics in connection with measurements are:
- The system on which a measurement is made has a definite value for the property being measured, even before the measurement is made.
- The instrument used for making the measurement does not affect the system; that is to say, it does not change the value of the property that is being measured.
Let us say we have a beaker full of water, and that we want to measure the temperature of the water. The system in this case is water, the property we want to measure is the temperature and the instrument we would use would of course be the thermometer. What the assumptions stated above imply are that firstly, the water in the beaker had a definite temperature before the thermometer was inserted into the water, and secondly, the thermometer when inserted into the water does not alter the temperature.
Thus, in Classical Physics, the physical properties of a system are attributes of the system that exist on their own, whether or not someone takes the trouble of measuring them.
|
 |
At the level of QM, it is impossible to measure a system without disturbing it |
|
The 'Rules' are Different in Quantum Mechanics
The point made above is extremely important because in QM the ball game changes drastically. Firstly, it is not possible to make definitive statements about the attributes of the system before any observation is made. Further, whenever one makes a measurement or an observation [as one says sometimes], it invariably disturbs the system.
It is important to note that it is not possible even in principle to reduce to zero the disturbance caused to the system observed, by the measurement process. If this disturbance could be reduced to zero, then it would mean that the measured quantity has a value independent of the measurement. QM rejects this view!
I hope you are able to appreciate the change in perspective that the advent of QM brought about. In Classical Physics, it was reasonable to suppose that a system had specific properties/attributes, whether or not it was observed. In QM, on the other hand, the attributes of a system made sense only when a measurement was made. In turn, this implied the existence of a measuring apparatus and an observer who carried out the measurement. Reality was thus related to the perception the observer had, based on the measurement he had carried out.
In the language used earlier, Classical Physics took an ontological view of reality, while QM took an epistemological view of reality. And as you can readily see, these are two fundamentally opposite points of view. As I mentioned before, though Einstein was responsible for pointing out for the first time that light had a wave-particle duality, that is it was quantum in nature, yet when QM flowered and took on a respectable mathematical shape, he did not like what seemed to be the underlying philosophy.
People who were pro-QM, said, “Look Prof, this thing works, it gives results, it gives a very good method of calculating quantities that we simply cannot calculate using Classical Physics. So this thing has got to be correct. How can you dismiss it?” Einstein’s reply was in effect,
“Maybe you are able to do some calculations and get correct results. However, I am not at all comfortable. How can one be sure that this mechanics would always deliver the goods? I personally believe one day or the other you would hit a road block and be forced to look for a new theory to replace what you call QM. You know why I distrust this QM? I shall tell you. It completely negates the picture of reality that Classical Physics gave us. You are telling me that reality is based on what one observes. Do you mean to tell me that the Moon does not exist if I do not observe it? That is pure nonsense!”
You get some idea of the tensions and the passions? What came out of it all was high drama, more thrilling than what you might expect in a Bollywood movie. All that, in the next instalment! Meanwhile, do not bite off all your nails please!
Dear reader, how do you like this series? Please share your impressions about this article by writing to h2h@radiosai.org mentioning your name and country. Thank you for your time.
Previous Articles In The Same Series |
|
|
|
|
|
|
|
|
|
|
|
|
|
|
|
|
|
|
|
|
|
|
